What can quantum many-body physics and optics bring to virology?
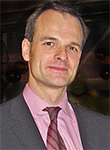
By Prof. Gabriel Aeppli, ETHZ, EPFL and PSI
Among the most important problems illuminated by the current COVID-19 pandemic are those of producing and measuring the immune response to the corona virus. The immune response defines the progression and lethality of the disease in an infected individual, while the idea of promoting such a response through a harmless trigger molecule or viral-like complex is at the core of vaccine development. A key challenge for both patient care as well as research is thus the metrology of the immune response. This entails measuring the antibodies which appear upon infection. Antibodies are proteins rather than nucleic acids, and can be clinically relevant even in small concentrations in the presence of many other proteins which are found in biological fluids, meaning that any meaningful assay must be highly sensitive and specific. Unlike the RNA which is the genetic material at the core of a virus, they cannot be chemically amplified using PCR (polymerase chain reaction) and subsequently identified, typically via fluorescence modulated by the presence or absence of complementary base pairs.
Five colleagues at University College London (John Hales, Paul Dalby and John Ward) and the Paul Scherrer Institute (Guy Matmon and myself) have recently published (//www.nature.com/articles/s41467-019-11604-z; a popular description oriented towards the biomedical community is //naturemicrobiologycommunity.nature.com/users/7272-ben-libberton/posts/53181-turning-viruses-into-lasers-could-revolutionise-hospital-diagnostics ) the results of experiments taking a physical rather than chemical approach to the problem of signal amplification in an immunoassay where we perform optical instead of chemical amplification; the research was conducted largely as the UCL PhD work of John Hales. What we are looking at here is a dye laser where the dye is a virus, created using the principles of synthetic biology, and the lasing is controllable by proteins which bind to the coat protein of the virus. The result is a mix and measure immunoassay with unprecedented sensitivity, and also a definite digital output – a medium is either lasing or it is not – which can be appreciated by anyone who has used a colorimetric test (e.g. a change from faint pink to red of a solution or paper) for a medical condition.
Figure 1a illustrates the basic ingredient of our viral laser, namely the M13 filamentary phage, which is genetically programmable quantum matter in the sense that the coat proteins and their assembly into an object, roughly 1 micron long and 6.5 nanometers in diameter, can be designed to include specific attachment sites for chromophores and target proteins such as particular antibodies, such as the monoclonal antibody shown in Figure 1b. For physicists and „top down“ nanoengineers, the size, complexity and reproducibility of the objects depicted in Figure 1, especially when compared to their „hard matter“ peers, namely quantum dots, should be remarkable. In our case, the (optically active) chromophore is fluorescein, a small molecule which has long been established as a laser dye freely suspended in solution. The virus in our case acts as a substrate for the dye, thus introducing a new environment which will define the radiative and non-radiative decay channels for the excited states populated during lasing.
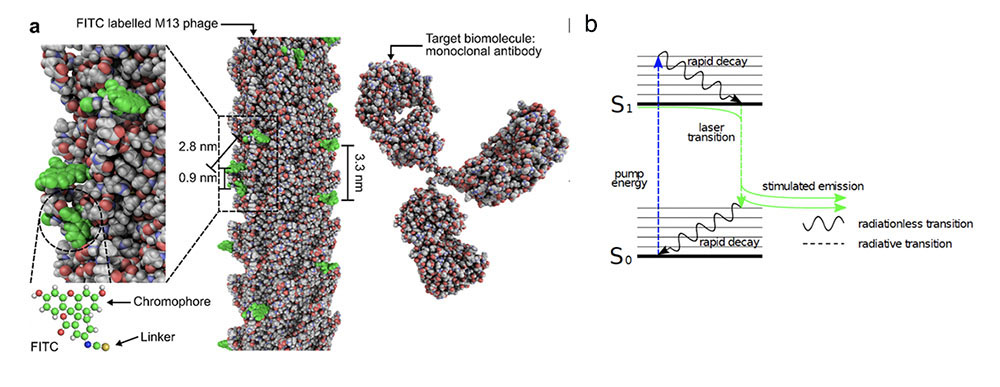
A laser consists of a medium, a pump and a resonator, and when we assembled these using our new viral dye, we were not disappointed in the sense that there was a clear lasing transition as a function of power and viral concentration. Figure 2 shows these data, as well as (orange) data points for free fluorescein in solution, with an optical density nearly matched to the optical density for the phage-immobilized chromophores at 351 pM/mL (blue points). Emission grows by many orders of magnitude as the lasing transition is crossed by small changes in pumping powers near threshold. The immobilization of the dye molecules clearly increases the lasing threshold, which finds a natural explanation in terms of non-radiative decay channels added on account of attachment to the viral substrates.
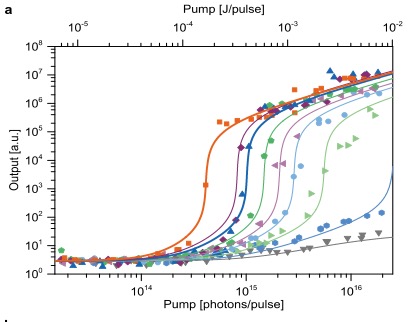
The emission curves as a function of pump power and dye concentration define a phase diagram very similar to that for equilibrium properties of matter familiar to MANEP members: as the concentration of dyes in solution is decreased the threshold curves move systematically to higher powers. The lines through the data correspond to a mean field theory analogous to that for the Landau theory of conventional phase transitions. Indeed, the lasing threshold obeys a Curie-Weiss-like law (Figure 3)
where K2 is the decay rate from the upper state of the dye, KF the strength of the coupling of the pump to the dyes, KL the loss rate of the resonator, KRAD the rate of spontaneous emission per dye into the resonator mode and D the number of dyes in the resonator volume. The parameter which is easiest to control here is D, which in turn implies that the analog of the Curie temperature is the ratio KL /KRAD of loss rates: as D approaches KL /KRAD from above, approaches infinity and lasing ceases.
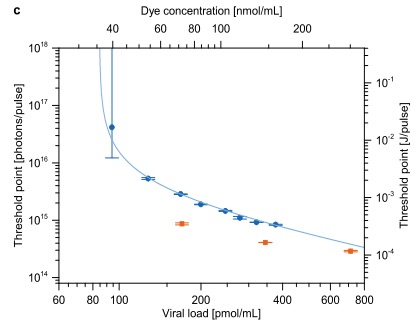
What Figure 3 shows is that by measuring the lasing threshold, we can obtain a well-defined value for D which depends only on knowledge of a single ratio (KL /KRAD ) and measurement of a strongly amplified optical signal, unlike the case of measuring fluorescence yield where there is a weak signal in the presence of large background, and calibration of output and input powers is necessary. We therefore provide a natural method for establishment of viral load, a quantity of very direct clinical interest because it determines the medical impact of an infection; for example, it has been noted that medical professionals are particularly susceptible to COVID-19 because of frequent and prolonged exposure to infected individuals. Of course, what we show here is a demonstration experiment with a synthetic virus, and one would need to establish a procedure whereby a natural virus such as COVID-19 would be brought into a lasing condition.
We began this article by presenting the immunoassay problem, and we therefore turn now to our demonstration that viral lasing is indeed modulated by the presence of antibodies. To enhance the performance of the assay, we have engineered a new laser with a new resonator (reduced mode volume), lower viral density in the solvent, to raise the odds that any given virus will encounter and stick to the target antibody, and higher density of chromophores per virus, to increase the effect of antibody docking on the optical response of a single virus as well as to compensate for the reduced viral density. Figure 4 shows the outcome in the form of optical yield versus pump power. We compare again unbound fluorescein with the viral laser, all in the presence or absence of the antibody. Without the antibody, there is again a significant shift between the thresholds, but in addition, while the rise beyond threshold is clearly non-linear and extending over two decades of output power, it is considerably attenuated relative to that for the unbound dye molecules. This is due to additional physics not already accounted for in the conventional theory of lasing; we have modelled this by taking into account (blue curve) the non-linearity of the optical response of the virus when it is subject to a strong optical field. Finally, we turn to what is of largest biomedical interest in Figure 4 – when antibody is added at the modest level of 90 fM/mL (green points), viral lasing ceases even while that of free fluorescein remains unaffected. Further experimentation suggests that we can detect, in the buffer solution used here, antibodies with concentrations as low as 30 fM/mL, which is several orders of magnitude smaller than what is obtained for ordinary biomolecular fluorescence.
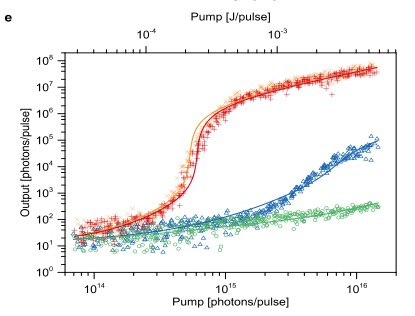
We have shown how a many-body quantum system interacting with photons in a resonator displays a phase diagram analogous to those for quantum matter at equilibrium. The quantum matter here is built using the self-assembly techniques of molecular biology rather than top-down nanofabrication or conventional crystal growth. While optical pumps, resonators and synthetic biology provide promising new tools for quantum matter research, our experiments also offer possible solutions to key challenges for virologists, namely those of measuring concentrations of antibodies and viruses in biological fluids.