EuCd2As2: A Magnetic Semiconductor
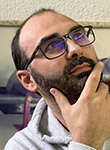
By David Santos-Cottin, UNIFR
Weyl semimetals are an exciting new group of materials, showing unique signatures in their transport and optical behavior, inherited from their distinct topological features. The presence of nodes in the electronic band structure of Weyl semimetals makes their electrons behave as if they are massless, and this leads to several interesting properties. EuCd2As2 was initially proposed as a magnetic Weyl semimetal based on density functional theory (DFT) calculations and experimental studies. In a magnetic Weyl semimetal, it would be possible to manipulate the topological properties using a small magnetic field. Without an external magnetic field, a small gap separates the conduction and valence bands. But in a magnetic field, the two bands overlap, creating a Weyl semimetal. In a broader context, the idea is to use the material’s magnetic structure to control its topology. However, a recent in-depth study, from an international research team, revealed that EuCd2As2 is not a Weyl semimetal but rather a magnetic semiconductor. This finding contradicts around 30 published papers asserting EuCd2As2‘s Weyl semimetal nature, emphasizing the need for caution when making conclusions, mostly based on first-principle calculations.
The key development in this detailed study involved synthesizing high-quality EuCd2As2 samples. To achieve cleaner crystals, their careful crystal synthesis used very pure starting materials, in particular, extremely clean europium. Various experimental techniques, including electronic transport, optical spectroscopy, and excited-state photoemission spectroscopy, were employed to determine the material’s ground state. The material was studied at various temperatures and using infrared spectroscopy under an external magnetic field up to 16 T (see figure). The consistent conclusion from all experiments was that EuCd2As2 behaves as a magnetic semiconductor – it combines antiferromagnetic behavior with activated electrical conductivity, and a band gap of 0.77 eV. An external magnetic field strongly impacts the band gap and the transport properties. Applying 2 T is enough to decrease the band gap by 125 meV.
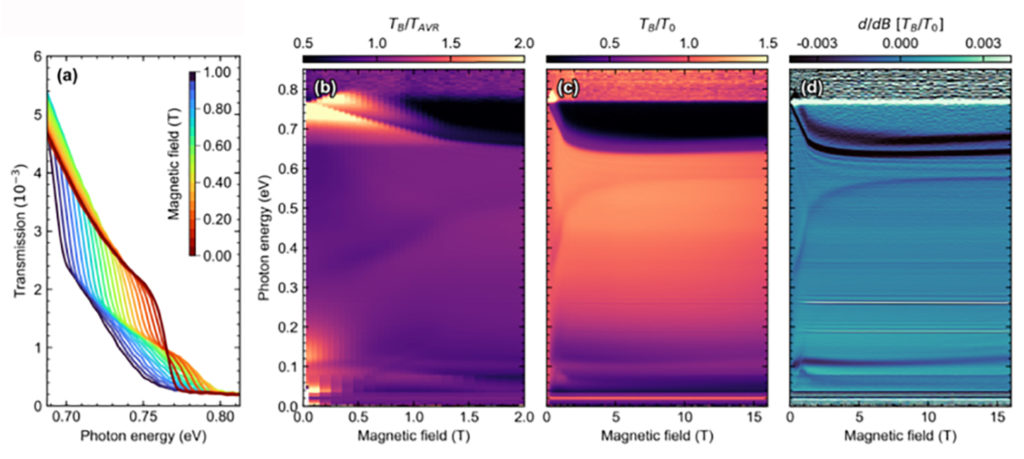
However, in contradiction to many previous studies, the material never ceases to behave as a semiconductor, even under a strong magnetic field. The coveted magnetic Weyl semimetal phase simply isn’t there. The main discrepancy in previous studies is attributed to europium’s f orbitals, causing strong electron-correlation effects that challenge DFT calculations. A noteworthy aspect is the almost forgotten power of magneto-optical spectroscopy, a technique that was widely used in the past to learn about semiconductors.
Based on article published in Physical Review Letters