A thermoelectric materials emulator (Science Magazine)
Converting heat directly into power represents a potential major source of renewable energy. An international group of physicists recently accomplished an experiment with cold atoms trapped by lasers at ETHZ which simulates this physical phenomenon. Experimental measurements were compared to theoretical predictions made at the University of Geneva, École Polytechnique and Collège de France in Paris, and the University of Bonn. This new approach may help to study in detail the mechanism of the thermoelectric effect and design new highly efficient materials. Results of this experiment have been published in Science.
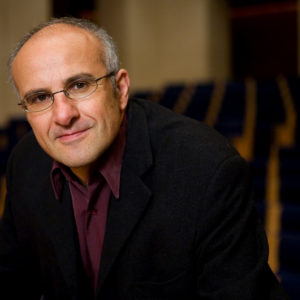
Thermoelectric effects were discovered almost two centuries ago, by Volta, Seebeck, and Peltier. They consist in a reversible coupling between heat and particle flow, which give rise for example to a finite voltage drop between the ends of an open-circuit conductor subject to a temperature gradient (Seebeck effect) or to the cooling or heating of a junction between two conductors in which a current is circulated (Peltier effect). These effects may for example provide a way to convert wasted heat into usable energy. Material physicists have been looking for efficient thermoelectric compounds [1], and a marked renewal of interest and research in this field has taken place since the mid-90’s [2]. Notwithstanding significant progress, the thermoelectric material commercially used today, Bismuth Telluride, is still the same one than five decades ago.
In a recent publication in Science [3], “thermoelectric” effects have been explored in an entirely different setting: that of ultra-cold atomic gases (see also [4]). Experimental measurements performed in the quantum optics group of the ETH-Zürich are compared to theoretical predictions made at the University of Geneva, École Polytechnique and Collège de France in Paris, and the University of Bonn. The experimental setup consists in two clouds of ultracold Lihtium atoms connected by a thin film creating a conducting channel. After heating one of the clouds the flow of heat and particles through the channel is measured. A large, transient flow of atoms from the hot cloud to the cold reveals a thermoelectric effect in the channel, a phenomenon never observed so far in a cold atom system.
The experiment can be modeled by combining a Landauer-Büttiker description of the transport through the channel with the known thermodynamics of the gas in the reservoirs, and excellent agreement between this theoretical description and the experimental results has been obtained. Thermoelectric effects in this context involve a competition between the dilatation of the reservoirs and the intrinsic thermopower of the channel. In the parameter range in which the experiments are performed, the thermoelectric effects are actually dominated by the properties of the channel.
Because the all the structures guiding the atoms are created by lasers, they can be widely tuned. By modifying the transverse confinement, the influence of the geometry of the channel on thermoelectric properties has been studied in this setup. Disorder can also be introduced in a controlled manner by shining a laser speckle into the channel, and transport can thus be tuned from the ballistic to the diffusive regime. In agreement with theoretical expectations, a stronger geometric confinement and, especially, a stronger disorder both lead to an increase of the thermoelectric effect.
The experiment can be viewed as the conversion of the initial temperature difference between the reservoirs into a particle current. Hence, a heat-engine is realized. Non-equilibrium thermodynamics can be used to evaluate the efficiency of this process. When formulated as an effective thermoelectric “figure of merit” ZT for the channel, as commonly done for thermoelectric materials, the resulting values are found to be quite large, typically in excess of ZT = 2, corresponding to a heat conversion efficiency approaching 30% of the Carnot value.
This work opens several perspectives and research directions at the crossroads of quantum optics, condensed-matter physics and non-equilibrium thermodynamics. Thanks to the controllability of ultra-cold atom experiments, the effect of geometry, interactions and disorder on the fundamental mechanisms at the origin of thermoelectricity can be explored. This may ultimately help in finding the necessary ingredients to design new efficient materials for thermoelectric applications. Thermoelectric effects may also prove useful to the field of ultra-cold atoms itself, by improving the cooling of these systems using the Peltier effect.
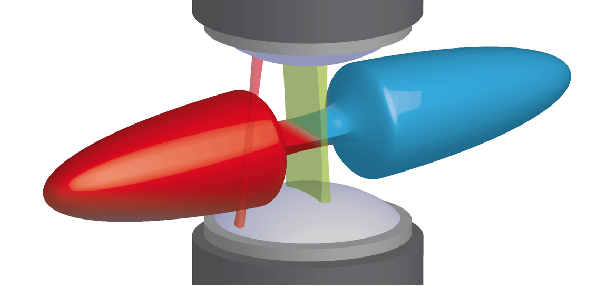
Figure : Principle of the experiment. Two clouds of cold Lithium atoms are connected by a thin quasi-two-dimensional channel. One cloud (in red) is heated by a laser beam, while a serapation (in green) prevents heat from flowing to the cold side. At the beginning of the experiment, the separation and the heating are removed and the flows of atoms and heat through the channel are measured. photo credit David Stadler, ETHZ
[1] For a recent review, see G.J. Snyder and E.S. Toberer, Complex Thermoelectric Materials, Nature Materials 7, 105 (2008)
[2] See e.g. J.P. Heremans, M.S. Dresselhaus, L.E. Bell and D.T. Morelli, When Thermoelectrics Reached the Nanoscale, Nature Nanotechnology 8, 471 (2013).
[3] J.P. Brantut, C. Grenier, J. Meineke, D. Stadler, S. Krinner, C. Kollath, T. Esslinger and A. Georges: A thermoelectric Heat Engine with Ultra-Cold Atoms, Science 342, 713 (2013)
[4] E.A. Hazlett, Li-Chung Ha and Cheng Chin, Anomalous thermoelectric transport in two-dimensional Bose gas, arXiv :1306.4018
Article published in
Science
Press release
in English